19.1 Electrochemical cells (AHL)
Written specifically for students to provide help and support for the IB Diploma chemistry programme this page provides full coverage of the syllabus content of Topic 19.1 Electrochemical cells (AHL). It encourages you to think critically and provides many questions with full worked answers so that you can monitor and improve your knowledge and understanding.
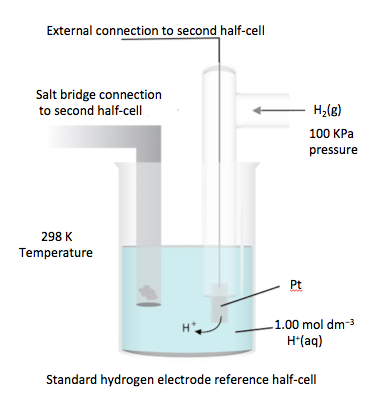
Learning outcomes
After studying this topic you should be able to:
Understand
Voltaic cells generate an electromotive force, EMF, resulting in the movement of electrons from the negative electrode (anode) to the positive electrode (cathode) through the external circuit. This EMF is known as the cell potential, E⦵.
- The standard hydrogen electrode, SHE, consists of an inert platinum electrode in a 1 mol dm-3 aqueous solution of hydrogen ions and in contact with hydrogen gas at 100 kPa and 298 K. E⦵ for the SHE is assigned the value of 0 Volts.
- The standard electrode potential, E⦵, of any half-cell is the potential (in volts) of the reduction half-equation under standard conditions (1.0 mol dm-3 concentration for solutions or 100 kPa for gases) measured relative to the SHE at 298 K.
- When aqueous solutions are electrolysed, water can be oxidized to oxygen at the positive electrode (anode) and reduced to hydrogen at the negative electrode (cathode).
- ΔG⦵ = − nFE⦵
When E⦵ is positive, ΔG⦵ is negative and the process is spontaneous. When E⦵ is negative, ΔG⦵ is positive and the process is non-spontaneous. When E⦵ is zero, then ΔG⦵ is also equal to zero. - The current, the duration of electrolysis and the charge on the ion affect the amount of products formed at the electrodes during electrolysis.
- Electroplating involves the coating of an object with a thin layer of a metal by electrolysis.
Apply your knowledge to:
- Calculate cell potentials using standard electrode potentials for half-cells.
- Use E⦵ values to predict whether a reaction is spontaneous or non-spontaneous.
- Determine standard free-energy changes, ΔG⦵, using standard electrode potentials.
- Explain the products formed during the electrolysis of aqueous solutions.
- Carry out laboratory experiments that could include single replacement reactions in aqueous solutions.
- Determine the relative amounts of products formed during electrolytic processes.
- Explain the process of electroplating.
Relationships & vocabulary
Nature of science
The use of standard electrode potentials, whose values are all relative to the standard hydrogen electrode, provides a good example of quantitative reasoning in science.
Scientists working together on electrochemical cell technologies, where they have had to consider the environmental and ethical implications of using fuel cells and microbial fuel cells, provides a good example of collaboration and ethical implications.
International-mindedness
Many of the world’s energy problems can be alleviated by using electrochemical cells as energy sources. Some cells, e.g. super-efficient microbial fuel cells, MFCs, (also known as biological fuel cells) can also help to clean-up the environment. The international community and national governments have to decide what to prioritise when it comes to funding research projects.
For more examples and links to International mindedness, Theory of knowledge, utilization etc. see separate page which covers all of Topics 9 & 19: Redox processes.
Vocabulary
standard hydrogen electrode, SHE | standard electrode potential, E![]() | electromotive force, EMF | cell potential |
charge (coulombs) = current (amps) x time (s) | electrolysis of 'water' | electroplating |
Learning slides
You can use this slide gallery for learning or for reviewing concepts and information. It covers all the key points in the syllabus for this sub-topic.
Something to think about
1. Voltaic cells and standard electrode potentials
This topic tends to cause students considerable difficulty when they come to answer questions on standard electrode potentials in Paper 2. It is also a topic that many teachers seem to find difficult - maybe there is some relationship between these two statements? The problem seems to stem from what is meant by "standard electrode potential". It could be that the IB data booklet does not help. For example, the lithium half-cell at the top of the electrochemical series in Section 24 of the data booklet appears as:
Because of the way it is written many students (and possibly teachers too) confuse this with an energetics equation and think that if they write the equation the other way round then E will be equal to + 3.04 V. This is the wrong way of looking at standard electrode potentials. It is better to consider the half-cell as lithium metal in contact with lithium ions, i.e. Li(s)/Li+(aq), and when this is connected to a standard hydrogen electrode the minus sign means that electrons will flow from this half-cell to the hydrogen half-cell and the voltage will be 3.04 V. The electrochemical series is simply a list of what happens when all the half-cells in question are connected in turn to a hydrogen half-cell. The only concept that is key to remember is that when any two half-cells are connected together to give a spontaneous reaction the electrons will flow from the more negative half-cell to the more positive half-cell and the difference between the two values will be the EMF (cell potential) for that cell.
Let's look at one example to illustrate this. What will happen spontaneously when an Fe2+(aq)/Fe3+(aq) half-cell is connected to an iodine half-cell, ½I2(s)/I–(aq)?
To solve this problem draw the cell diagram and write the E values from the data booklet under the relevant half-cells:
½I2(s)/I−(aq) || Fe2+(aq)/Fe3+(aq)
+ 0.54 V + 0.77 V
The more negative (actually the least positive) is the iodine half-cell so the electrons will flow from this half-cell to the Fe2+(aq)/Fe3+(aq) half-cell. That is, the iodine half-cell will lose electrons and the iron ion half-cell will gain electrons so the two half-equations will be:
Fe3+(aq) + e– → Fe2+(aq)
2I–aq) → I2(s) + 2e–
So the overall spontaneous reaction is that iron(III) ions will oxidize iodide ions to iodine. The total cell potential (EMF) for this reaction will be 0.23 V.
2Fe3+(aq) + 2I–aq) → I2(s) + 2Fe2+(aq) Ecell = 0.23 V
A similar question could be asked a different way. Will iron(III) ions oxidise bromide ions to bromine? Solve it the same way. Write the two half-cells, look up their standard electrode potentials and then determine which will be the spontaneous reaction.
Fe2+(aq)/Fe3+(aq) || ½Br2(l)/Br–(aq)
+ 0.77 V + 1.07 V
Now the iron ion half-cell has the more negative (less positive) value so the electrons flow from this half-cell to the bromine half-cell. The half-equations for the spontaneous reactions become:
Fe2+(aq) → Fe3+(aq) + e–
Br2(l) + 2e– → 2Br–aq)
The overall equation for the spontaneous reaction will be:
2Fe2+(aq) + Br2(l) → 2Br–(aq) + 2Fe3+(aq) Ecell = 0.30 V
What this tells us is that iron(III) ions will not spontaneously oxidize bromide ions to bromine. In fact bromine will spontaneously oxidize iron(II) ions to iron(III) ions.
If all this is understood you should now be able to answer any questions thrown at you on the use of standard electrode potentials. I have written a separate page under Areas of difficulty for Paper 2 which gives some more examples.
One question that students sometimes ask is how the standard electrode potential of very reactive metals like sodium can be determined. You might find it interesting to read a research paper written by Edgar Reynolds Smith and John Keenan Taylor in 1940 which details how they obtained the value for the sodium half-cell. Essentially they made use of an indirect method which depends on firstly measuring the potential of dilute sodium amalgam with respect to an aqueous solution of its ions and a reference electrode, and secondly, the difference in potential between the sodium amalgam and the pure metal when both are immersed in a non-aqueous conducting solution containing sodium ions. This non-aqueous solvent must not react with sodium and must dissolve the alkali salt sufficiently to form an electrolytic solution.
2. Electrolysis of aqueous solutions
This is quite a difficult topic to learn well. The trick is to understand that only certain examples are required to illustrate the various factors that affect the products (and their amounts) produced during electrolysis.
Position in the electrochemical series. This is almost completely limited to the position of metals and their ions and, of course, hydrogen and water. The obvious examples to include here are the electrolysis of dilute sodium hydroxide solution which behaves as 'water' (as hydrogen is evolved in preference to sodium) and the electrolysis of copper(II) sulfate solution with inert electrodes (where copper is deposited in preference to hydrogen ions gaining electrons). The position of negative ions is not really required by the IB as under normal conditions hydroxide ions will give up electrons in preference to other anions. The obvious example of this is the electrolysis of 'water' using dilute sulfuric acid. It is nice to see this using a Hofmann voltameter (right) although this particular piece of apparatus is not on the syllabus as such.
Nature of the electrode. For the most part electrodes made of graphite or platinum are inert and play no part in the products. The classic examples where they do affect the products are the use of copper electrodes in the electrolysis of copper(II) sulfate and the now almost obsolete mercury cell once used in industry where a flowing cathode of mercury results in a sodium amalgam product.
Concentration of the electrolyte. The classic example here is sodium chloride solution. For dilute solutions the products are almost exclusively hydrogen and oxygen but as the solution becomes more concentrated chlorine rather than oxygen is evolved at the positive electrode (anode) although a mixture containing both gases is actually formed. This is fertile ground for many a chemistry Extended Essay and could perhaps also be used for the individual scientific investigation.
Relative amounts of products. This is the application of Faraday's Laws although the laws themselves are not required. What this does mean is required is the concept of connecting two electrolysis cells in series. This way the same amount of electricity must be passing through both cells and if the amount of one product is known the amount of other products can be deduced from their relevant half-equations.
Electroplating. Examples here include the refining of copper using a small piece of pure copper as the negative electrode and impure copper the positive electrode (although the use of that lovely phrase 'anodic sludge' is not required) and plating with metals such as chromium where the object to be plated is made the negative electrode (cathode).
Test your understanding of this topic
(Note that your teacher may have restricted your access to some or all of these questions and worked answers if they are going to use them as a class test or set them as an assignment.)
For ten 'quiz' multiple choice questions with the answers explained see MC test: Electrochemical cells (AHL).
For short-answer questions on voltaic cells see Electrochemical cells (1) (AHL) questions and for a second set of short-answer questions on electrolytic cells see Electrochemical cells (2) (AHL) questions.
More resources
1. A useful video describing the standard hydrogen electrode. Note however that although written on the screen there is no verbal inclusion of the need for the temperature to be 25 oC (298 K) for standard conditions. Also the hydrogen gas shown must actually be slightly higher than the required one atmosphere pressure as it bubbles out from below the surface of the solution.
2. A good video on the electrolysis of concentrated sodium chloride solution which explains it well.
3. The electrolysis of 'water' using dilute sulfuric acid and a Hofmann voltameter. It clearly show the volume relationship and the identity of the two gases.